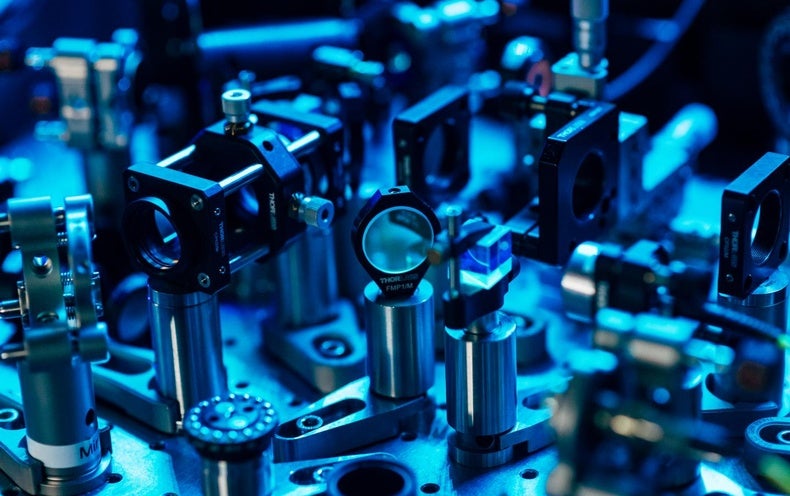
Quantum cryptography promises a future in which computers communicate with one another over ultrasecure links using the razzle-dazzle of quantum physics. But scaling up the breakthroughs in research labs to networks with a large number of nodes has proved difficult. Now an international team of researchers has built a scalable city-wide quantum network to share keys for encrypting messages.
The network can grow in size without incurring an unreasonable escalation in the costs of expensive quantum hardware. Also this system does not require any node to be trustworthy, thus removing any security-sapping weak links.
“We have tested it both in the laboratory and in deployed fibers across the city of Bristol” in England, says Siddarth Koduru Joshi of the University of Bristol. He and his colleagues demonstrated their ideas using a quantum network with eight nodes in which the most distant nodes were 17 kilometers apart, as measured by the length of the optical fiber connecting them. The team’s findings appeared in Science Advances on September 2.
Two’s Company, Three’s a Crowd
Quantum cryptography involves using the laws of quantum physics to create a private key for encoding and decoding messages, a process called quantum key distribution, or QKD.
In the most often used protocol for QKD, one party, Alice, prepares and sends a quantum bit, or qubit, to another, Bob. The qubit is a particle in a quantum superposition of two states. Bob chooses, at random, one of a set of measurements of the particle. If Bob chooses the correct type of measurement, he will know the value Alice encoded in the qubit. After a sequence of measurements of such qubits, Alice and Bob publicly exchange notes and agree to use the results of a certain subset of Bob’s measurements (both know the value of each qubit for this subset). They discard the other measurements. Crucially, the results are not public, and Alice and Bob use them to create a private key to encrypt and decrypt messages sent over a public link.
But this method is hard to scale up. Imagine you want to add another user, Charlie, to the network. One option is for Bob and Charlie to establish a secure link. Then Alice can send a message to Charlie via Bob, but she has to trust Bob.
“That’s not very attractive,” says team member Sebastian Neumann of the Institute for Quantum Optics and Quantum Information–Vienna. “The whole point of quantum cryptography is unconditional security.”
To avoid having to trust Bob, Charlie can connect directly to both Alice and Bob. Now those two will need extra hardware to communicate with Charlie because a new node cannot be added without disrupting the existingnodes. And this issue arises when you add just one extra node. The requirements mushroom in cost and complexity as new nodes are added. For example, a two-node network has one link, a three-node network has three of them, an eight-node network has 28, and a 100-node network needs 4,950.
Joshi and his colleagues used another QKD protocol that involves sharing entangled particles between any two nodes to design a new kind of network that overcomes many of these problems.
A Metropolitan Multiplex
In this protocol, Alice and Bob use pairs of entangled photons to create a private key. Given one photon from such a pair, Alice randomly performs one of a certain set of measurements. Bob does the same with his particle. Because the photons are entangled, Alice and Bob will get the same result if they make the same measurement. The two publicly share their sequence of measurements of the paired particles. They then choose the subset that would have given them the same results and discard the others. These results, which are never publicly disclosed, form the basis for a private key.
Instead of building a network in which each of the eight nodes is physically connected to all the other nodes, the researchers created one with a central source that sends entangled photons to the eight nodes, named Alice, Bob, Chloe, Dave, Feng, Gopi, Heidi and Ivan. Each node is only connected via a single optical fiber link to the source, making a total of eight links—far less than the 28 that would be required for traditional QKD with no trusted nodes.
So even though the nodes are not physically connected, the protocol the researchers developed establishes a virtual link between each pair of them via the magic of quantum entanglement such that each pair can create a private key.
The central source has a so-called nonlinear crystal that spits out a pair of photons that are entangled in their polarization. These photons have a wavelength centered on roughly about 1,550 nanometers, plus or minus a few tens of nanometers. If one were to look for and find one of the photons at, say, 1,560 nanometers, the the law of conservation of energy dictates that its entangled partner would have a wavelength of 1,540 nanometers. One such narrow wavelength is a channel. The central source splits up the wavelength of the initial entangled pair into 16 channels, eight on either side of, and equidistant from, 1,550 nanometers. Doing so effectively creates eight pairs of channels, numbered 1 to 8 on one side and –1 to –8 on the other. Upon measurement, the photons will, of course, be found in only a single pair of channels upon measurement, and not in the others.
These channels are then combined, or multiplexed, in the same optical fiber and sent to each node. Each node gets a different combination of channels. For example, Alice received channels 2, 6, 7 and 8; Dave receives –6, –4, –3 and 1; and Gopi receives –8, 5, 4 and –2. The wavelengths are chosen such that any two nodes always share at least one pair of channels that could have entangled photons in them. In the above scheme, Alice and Dave share channels 6 and –6; Alice and Gopi share channels 2 and –2 and 8 and –8; and Dave and Gopi share –4 and 4.
Each node monitors all of its channels to measure the polarization state of a potential photon if it appears in one of them. So if a photon detector were to click for Alice, she effectively has made a measurement to see whether the photon is polarized in one direction (horizontal or vertical) or another (diagonal or antidiagonal). As it happens, only one of the other nodes would have detected the corresponding entangled photon at exactly the same time.
After a series of such measurements with a large number of pairs of entangled photons, the nodes broadcast their photon counts and the corresponding time stamps of when the photons were detected. This information allows the nodes to figure out which pairs of photons were measured by which pair of nodes. For example, Alice and Dave realize that they have a strong correlation in the number of detections for a certain time stamp. This correlation represents their share of entangled photons. Alice and Dave can now use the results of these measurements to establish a key between the two of them.
Scaling Up Securely
Adding a new node is simple: just connect it to the central source, which only has to modify its channel-splitting-and-multiplexing scheme. But none of the existing nodes need worry. “Alice does not have to change anything as the network changes,” Joshi says.
Also, the additional hardware required scales linearly with the increase in nodes—a major improvement, compared with earlier techniques. Crucially, none of the nodes have to be trusted, yet any pair of them can establish a secure link to create an unbreakable quantum key, which can be used to encode and decode messages.
Future large-scale quantum networks will have to solve at least two major problems: One is that they must interconnect an arbitrarily large number of users. Secondly, such networks have to span vast intracontinental and intercontinental distances—something that requires using either quantum repeaters to extend the range over which one can distribute quantum states or satellites to beam down qubits or entangled particles to nodes on the ground.
Ronald Hanson of Delft University of Technology in the Netherlands, who was not involved in the new work, acknowledges that it extends QKD to “reach many more users within the limited range of QKD without repeaters.”
Joshi’s team admits that its work has not yet tackled the issue of distances larger than the span of a small city. To increase the range, the researchers are thinking of using satellites to carry their central source of entangled photons. “We are working toward making such a source space compatible,” Joshi says. “We have to make it robust enough.”
"network" - Google News
September 03, 2020 at 05:45PM
https://ift.tt/2DosukH
Physicists Create City-Sized Ultrasecure Quantum Network - Scientific American
"network" - Google News
https://ift.tt/2v9ojEM
https://ift.tt/2KVQLik
Bagikan Berita Ini
0 Response to "Physicists Create City-Sized Ultrasecure Quantum Network - Scientific American"
Post a Comment